Basic Sciences
KEY POINTS
- Ionising radiation is a form of energy that has therapeutic as well as potentially harmful biological effects.
- Clinical use of radiation should be limited to doses as low as reasonably achievable to accomplish clinical objective.
- Personal safety measures include hazard awareness, maintenance of distance from the source of radiation, the use of shielding, and developing protocols to prevent and manage radiological accidents.
- Dosimeters can be used to estimate the personal cumulative radiation exposure. Avoid exceeding the recommended limit at your organisation.
- Particular attention should be made to minimise exposure to at-risk individuals such as pregnant women, children, and those subjected to repeated exposures.
INTRODUCTION
Ionising radiation is electromagnetic wave-particle energy that is sufficiently powerful to effect changes to atoms. Such energy can be found in healthcare settings and has the potential to cause biological effects.
With an ever-increasing number of radiological procedures being performed, anaesthetists, other healthcare workers, patients, and the public may be inadvertently exposed to ionising radiation. Ionising radiation has the potential for harm, which may arise in a deterministic (dose-related) or stochastic (random) manner. Examples of radiation-induced harm include burns, cellular apoptosis, cancer, and heritable genetic mutations.
Despite its importance, awareness of the risk of ionising radiation to biological systems is often lacking amongst doctors.1,2 This is partly attributed to our inability to perceive the presence of ionising radiation. It is arguable as to what constitutes a safe dose but in order to minimise risk, we need to minimise unnecessary radiation exposure.
Three principles guide safe use of radiation: (1) intended benefits of clinical radiation should outweigh the potential for harm, (2) radiation should be limited to ‘‘as low as reasonably achievable/practicable’’ doses required to achieve clinical objective,3 and (3) personal and institutional safety measures should be followed to mitigate potential harm from radiation.
What is Ionising Radiation?
Ionising radiation is energy released from an atomic source in the form of electromagnetic radiation, such as x-rays, gamma rays, and ultraviolet radiation or particle emission, for example, alpha, beta, and neutron particles. Ionising radiation travels from its source up to the speed of light, but it is not readily perceptible to our senses.
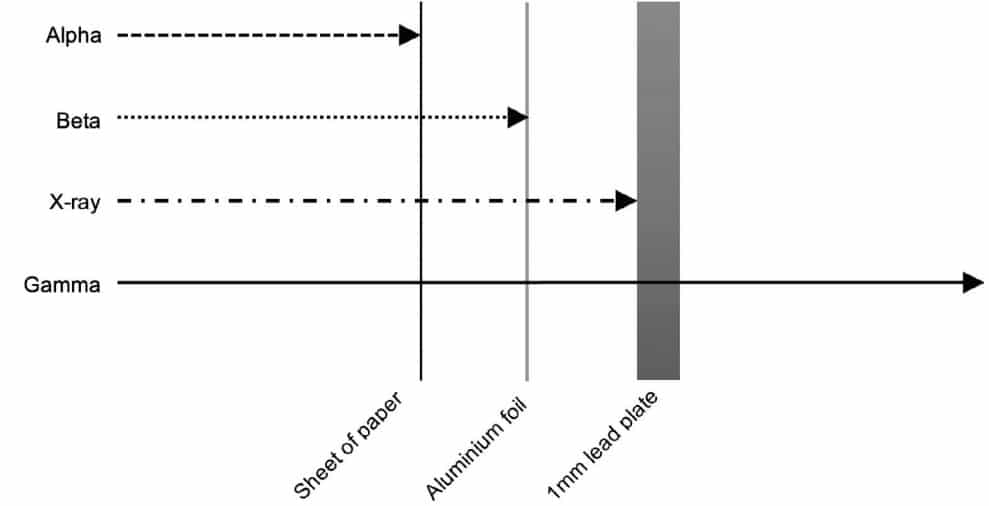
Figure 1. Penetration of various forms of ionising radiation.
It may pass through matter unimpeded or it may interact with matter at the subatomic level causing changes to atomic electron shell configurations and physicochemical properties. The ability of radiation to pass through matter depends on its nature, as illustrated in Figure 1. It is the interaction between ionising radiation and matter that has given it remarkable clinical utility but is also the reason radiation is a potential health hazard.
Radiation exists throughout the environment. Natural sources surround us, such as the cosmic radiation from space, radon gas released from ground rock, and even in radioactive substances in the water and foods we consume. The average annual background radiation dose we receive is approximately 2 to 3 mSv.4,5 Of particular concern is artificial radiation in the workplace, such as that in medicine and industry, as it is typically of a greater effective dose, more frequently encountered, and hence potentially more harmful.
Radiobiology and Hazards of Ionising Radiation
Radiobiology is the study of radiation and its effects on biological systems,6 which can broadly be divided into somatic and genetic or hereditary effects. Harm from radiation is dependent on the type of radiation, dosage, duration of exposure, and the susceptibility of the tissue to damage. The mechanisms of damage may be direct, such as direct ionisation of DNA interfering with its replication, or indirect, such as radiolysis of water, causing the formation of reactive species.4,6 These events can lead to cellular apoptosis, gene mutations, and the formation of cancer.
Much of the data regarding radiation effects is derived from experimental and epidemiological studies and case reports. Exposure to radiation may produce dose-dependent effects (deterministic effects) or a predisposition to effects by chance (stochastic effects). Dose-related harm occurs at a cellular level and manifest as radiation burns, cell death, and tissue necrosis. The main stochastic effect is the development of cancer, which, based on a study of nuclear industry workers, suggests a linear association between the development of cancers and cumulative radiation dose.7
Table 1 shows a simplified catalogue of procedural radiation doses, which will vary with the patient, equipment, and protocols in use. For perspective, the average annual environmental radiation dose we receive is typically around 2 to 3mSv. One sievert (Sv) would produce symptoms of radiation sickness, and a lethal dose of radiation is considered to be a median of 3.5 Sv, causing death to 50% of the exposed population within 60 days.10
Quantification of Ionising Radiation
The absorbed dose is the energy absorbed by matter from radiation and is a basic physical measurement of radiation exposure, the physical unit being the Gray (Gy):
1 Gy = 1 joule of radiation energy per kilogram of matter (Jkg–1) .
This is the physical dose. The clinical limitation of using this unit is that it does not account for the different radio-sensitivities of tissue and the biological effects of the various types of ionising radiation.
The equivalent dose (unit: Sv, Jkg–1) is used to describe the biological effect of radiation on an organ or tissue type.7 A weighting factor is used to account for the different types of radiation and their differing toxicity on tissues. This is an estimated equivalent dose.
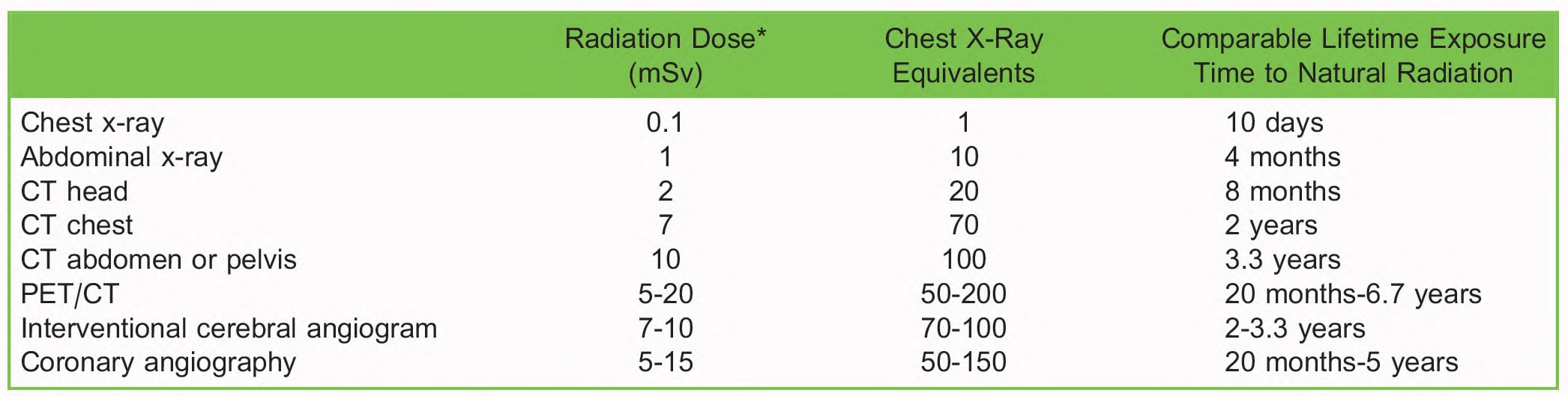
Table 1. Effective Radiation Dose of Common Radiological Procedures in Adults. CT indicates computed tomography; PET, positron emission tomography. *Approximate effective dose. There is a wide variation given the equipment used, the type of study performed, and the patient characteristics4,8,9
For example, the weighting factor of x-rays used in diagnostic radiology is 1. Nuclear medicine and specialised cancer treatment hospitals may utilise gamma and beta radiation (weighting factor 1) in the clinical setting or even alphaparticle radiation (weighting factor 20). An area of skin exposed to 1 Gy of x-ray or beta-radiation would have received an equivalent dose of 1 Sv. If the same area of skin receives 1 Gy of alpha-particle radiation, the equivalent dose would be 20 Sv, reflecting greater potential harm. The weighting factor is an approximation.
The effective dose is the effect of radiation on the whole body, which is the sum of the equivalent doses to each of these various tissues of the body. If the body is exposed to a mixture of radiation types, the equivalent dose would be calculated for each in order to arrive at the effective dose. Figure 2 shows the relationship between the physically absorbed dose, equivalent dose and effective dose of radiation.
Sources of Medical Radiation
For anaesthetists, the more frequently encountered forms of radiation, such as x-rays, are electronically generated and may be activated and deactivated electrically, thereby controlling the emitted dose. Other artificial sources include radioisotopes and radiopharmaceuticals, which constantly emit radiation through radioactive decay and cannot be deactivated as readily. Instead, they are produced by nuclear reactions and rely on containment in shielded vessels for safe transfer and use.
The effective dose from these radiopharmaceuticals varies with the type of radiation from the source, duration of exposure, distance, and tissue target. It also depends whether radiation exposure was external or internalised, such as in a body cavity, ingested, or inhaled.
Examples of radiopharmaceuticals include sestamibi, which is used for cardiac imaging; iridium-192, which is used in brachytherapy; technetium-99m, which is commonly used in nuclear imaging; and iodine-131, which used in the treatment of
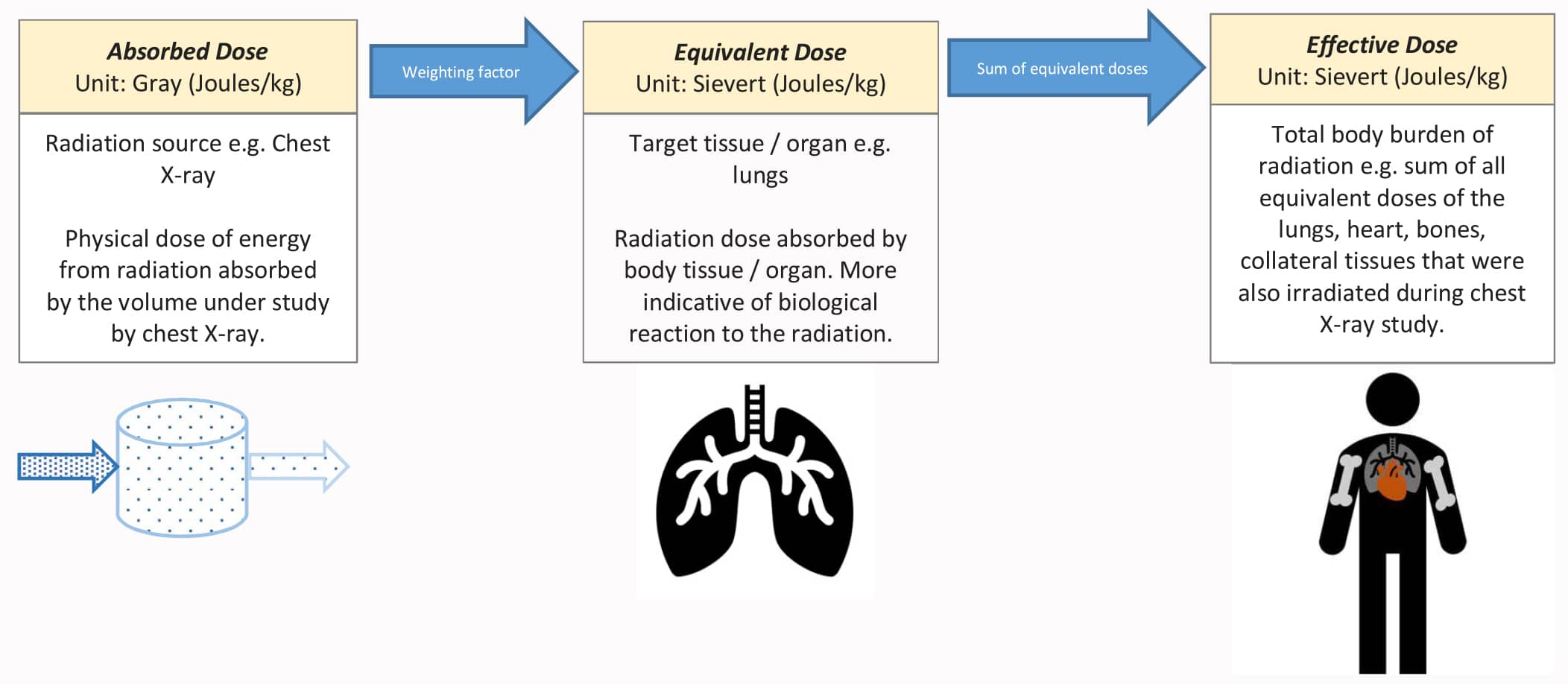
Figure 2. The relationship between the physically absorbed dose, equivalent dose and effective dose of radiation.
thyroid disease. These radioactive materials carry the risk of contaminating the environment and people. They should be used in controlled environments by specifically trained personnel.
IONISING RADIATION AND THE ANAESTHETIST
Potential sources of exposure to ionising radiation include the following:
- Operating theatre: use of x-ray in orthopaedics or fluoroscopy in vascular surgery;
- Interventional radiology suite: for acute stroke thrombectomy service;
- Diagnostic radiology: sedating a paediatric patient for a computed tomography scan;
- Endoscopy suite: endoscopic retrograde cholangiopancreatography;
- Nuclear medicine department: sedation or anaesthesia for brachytherapy or external beam radiotherapy;
- Accident and emergency treatment: resuscitating patients with radiological contamination.
Radiation safety is subject to national regulation, such as the United Kingdom’s Ionising Radiation (Medical Exposure) Regulations.11 The guidelines and recommendations are based on data from international authorities such as the International Commission on Radiological Protection or the International Atomic Energy Agency. The implementation of these regulations is coordinated at an institutional level by a radiation protection advisor or medical physicist.
Radiation safety is ultimately determined at the personnel level and stakeholders, such as anaesthetists, need to contribute to a culture of safety. We do so by maintaining close communication with our multidisciplinary team when engaging with a radiological procedure. In doing so, we can take appropriate protective measures during episodes of high radiation utilisation, such as fluoroscopic angiography during vascular procedures.12
A wearable dosimeter is recommended for staff, including anaesthetists who frequently work in such settings, to help alert the individual of accumulating radiation exposure. There are various types including film-badge, thermoluminescent, and electronic detectors.
GENERAL SAFETY MEASURES
A risk-benefit assessment must be made prior to exposing patients to medical radiation. At-risk groups requiring special considerations include child-bearing-age or pregnant women, children, and those with a history of high cumulative radiation exposure.
It is suggested that the whole-body effective dose of occupational radiation exposure should not exceed 20 mSv per year, averaged over 5 years with a maximum of 50 mSv in a single year,13 and the developing foetus should not receive more than 1 mSv of radiation in addition to natural background radiation.
Training and Education
A safe approach to radiation use requires education and training of the personnel involved and is typically coordinated by a radiation protection advisor. Protocols should be in place to help guide action during major incidents such as fire, power failure, and radiation leak, particularly when working in a nuclear medicine department. Drills should be carried out to practice management of clinical staff, patients, and the public in these scenarios.
If anaesthesia is to be conducted for such radiotherapy suites, it is recommended to develop a protocol and an evacuation safety drill. This measure is to anticipate the need to remove a patient from an out-of-control radiation source, for example spillage of a radiopharmaceutical or failure of a brachytherapy device.
Environment and Equipment
A suitable environment should be available for the use of radiation to limit unnecessary exposure to others. Often, these areas are constructed with shielding in the walls, floors, and ceilings, and incorporated into openings such as doors. In our hospital, the maximum allowable radiation escaping these restricted areas is limited to 3 mSv for x-ray areas and 1 mSv for nuclear medicine areas. These areas should have restricted access to unauthorised personnel, with prominent visual or auditory warnings of radiation. In many instances, these sites may be remote to the operating theatre facilities.
The higher energy ionising radiation used in radiotherapy suites mean the anaesthetised patient is typically monitored out of line-of-sight, often at a distance within a heavily shielded control room. There should be the facility for telemetry of the physiological monitor, as well as cameras to allow visual monitoring of the patient. Close communication should be maintained with the radiotherapy operator, particularly in the event that rapid access is required to the patient. Such dense shielding may limit the effectiveness of mobile communications equipment.
Appropriate protective equipment should be used, for example 0.35- to 0.5-mm-thick lead apron or equivalent, thyroid shield, goggles, and caps to protect radiosensitive organs when working with x-rays.12 Dosimeters should be issued to personnel regularly exposed to radiation to monitor for excess cumulative dose. Other devices that could be used for protection include mobile x-ray barriers or glass screens.
Distance
Radiation behaves according to the inverse square law, in that intensity is inversely proportional to the square of the distance from the source. Therefore, at 2 m distance from a radiation source, one would be subject to one-fourth of the radiation dose in comparison to being 1 m from the source. At 4 m, the radiation intensity would be 1/16 of that at 1 m. Measures to distance the anaesthetist from the radiation source whilst caring for an anaesthetised patient include the use of remote monitoring and equipping the anaesthetic machine with extended breathing circuits and infusion pump tubing. A shielded control room from which to monitor the anaesthesia could provide optimal protection from direct and scattered radiation.
SPECIAL CONSIDERATIONS
Radiological hazards may present as industrial, military, or terrorism-related incidents and may involve mass casualties.10 It is imperative to liaise with the relevant authorities, such as the fire department, to coordinate decontamination of the casualties as they may continue to emit radiation, posing a hazard to others. If the clinical care of such patients may potentially be expected at your locale, contingency planning for such incidents should be in place.
Exposed individuals can experience symptoms of acute radiation toxicity, especially if the dose was especially high (ie, approaching 1Gy).14 Initial symptoms may be nonspecific, such as generalised malaise. Specific syndromes are possible and related to which organ systems are affected. Ingested radioactive material may result in a gastrointestinal syndrome, whereby mucosal disruption may produce signs and symptoms such as nausea, vomiting, diarrhoea, and electrolyte disturbance. Other recognised patterns of acute toxicity may affect the cutaneous, haematological, and cerebrovascular systems and if severe, may lead to multi-organ failure.
The treatment goal in the setting of acute radiation syndrome is to remove the source by decontamination or distancing, providing supportive therapy and administration of antidote if appropriate. Just as there are radio-sensitising drugs used to enhance the cellular effects of radiation, radio-protective drugs, such as aminofostine,15 exist to mitigate adverse effects, typically acting as free radical scavengers. Some drugs such as Prussian blue are used as antidotes for caesium or thallium poisoning, and potassium iodide is used for radioactive iodine ingestion.10
Supportive therapy also includes management of symptoms with fluids, antiemetics, antibiotics to stave off opportunistic infections, and parenteral nutrition therapy. Granulocyte colony stimulating factor, erythropoietin, and haematopoietic cell transplants have been used to sustain haematopoiesis.14 Surveillance, in the form of blood tests to monitor for leukopenia and electrolyte disturbance, will help guide these supportive treatments.
SUMMARY
Advances in radiology and nuclear medicine bring numerous diagnostic and therapeutic benefits to the clinical management of patients. As such procedures become more common, it requires healthcare professionals to be cognizant of the risks and dangers. The World Health Organisation cites healthcare as the main source of artificial radiation exposure to the public and so clinicians have to be accountable in its use.
With responsible practice, healthcare professionals are unlikely to exceed the recommended limits for radiation exposure or to suffer from acute effects of radiation toxicity.12 To that end, we continue to have a shared responsibility with our multidisciplinary colleagues to protect patients, personnel, and the public from the potential harm of ionising radiation.
REFERENCES
- Shiralkar S, Rennie A, Snow M, Galland RB, Lewis MH, Gower-Thomas K. Doctors’ knowledge of radiation exposure: questionnaire study. BMJ. 2003;327(7411):371-372.
- Dauda AM, Ozoh JO, Towobola OA. Medical doctors’ awareness of radiation exposure in diagnostic radiology investigations in a South African academic institution. SA J Radiol. 2019;23(1):1707. doi:10.4102/sajr.v23i1.1707
- Valentin J. ICRP publication 105: radiological protection in medicine. An. ICRP 37 (6). 2007. https://journals.sagepub.com/ doi/pdf/10.1177/ANIB_37_6
- Lin EC. Radiation risk from medical imaging. Mayo Clin Proc. 2010;85(12):1142-1146. doi:10.4065/mcp.2010.0260
- Taylor J, Chandramohan M, Simpson KH. Radiation safety for anaesthetists. Contin Educ Anaesth Crit Care Pain 2013;13(2):59-62.
- Suntharalingam N, Podgorsak EB, Hendry JH. Basic radiobiology. In: Podgorsak EB, ed. Radiation Oncology Physics: A Handbook for Teachers and Students. Vienna: IAEA publication; 2005:485-508.
- Richardson DB, Cardis E, Daniels RD, et al. Risk of cancer from occupational exposure to ionising radiation: retrospective cohort study of workers in France, the United Kingdom, and the United States (INWORKS). BMJ. 2015;351. doi: https:// doi.org/10.1136/bmj.h5359
- Patel S, Reddy U. Anaesthesia for interventional neuroradiology. BJA Educ. 2016;16(5):147-152.
- Mettler FA Jr, Huda W, Yoshizumi TT, Mahesh M. Effective doses in radiology and diagnostic nuclear medicine: a catalog. Radiology. 2008;248(1):254-263.
- Hagby M, Goldberg A, Becker S, Schwartz D, Bar-Dayan Y. Health implications of radiological terrorism: perspectives from Israel. J Emerg Trauma Shock. 2009;2(2):117-123.
- British Institute of Radiology, Society and College of Radiographers and The Royal College of Radiologists. A Guide to Understanding the Implications of the Ionising Radiation (Medical Exposure) Regulations in Diagnostic and Interventional Radiology. London: The Royal College of Radiologists; 2015.
- Ho P, Cheng SWK, Wu PM, et al. Ionizing radiation absorption of vascular surgeons during endovascular procedures. J Vasc Surg. 2007;46(3):455-459.
- International Atomic Energy Agency. Radiation Protection and Safety in Medical Uses of Ionizing Radiation, IAEA Safety Standards Series No. SSG-46. Vienna: IAEA; 2018.
- Lo´pez M, Martı´n M. Medical management of the acute radiation syndrome. Rep Pract Oncol Radiother. 2011;16(4):138- 146.
- Kouvaris JR, Kouloulias VE, Vlahos LJ. Amifostine: the first selective-target and broad-spectrum radioprotector. Oncologist. 2007;12(6):738-747. doi:10.1634/theoncologist.12-6-738